Noise, often avoided by artists and engineers in traditional music, can be creatively harnessed in a variety of ways. In synthesis, it can be used as a control source: it is often used in conjunction with a type of circuit called a sample and hold to create random voltages (this is covered more in depth in a previous article on randomness, which can be read here). It can also be used to create percussive sounds such as cymbals, hi-hats, and snares. Noise can be used in sound design applications to mimic the sound of wind and the lap of waves. Additionally, it can be used to thicken up and add sonic interest to harmonically simple waveforms.
So what is noise and how has it been used historically? First, let's explore the different kinds of noise.
Flat Noise: White and Grey Noise
The names of the different types of noise are partly derived from an analogy to visible light. This started with white noise, as it was originally thought to share a common energy distribution with white light. As other types of noise were classified, they followed the same naming convention. The comparison of noise to light isn't entirely accurate, but it gives a good idea of the makeup of the different types of noise and how they are related to different spectrums of energy.
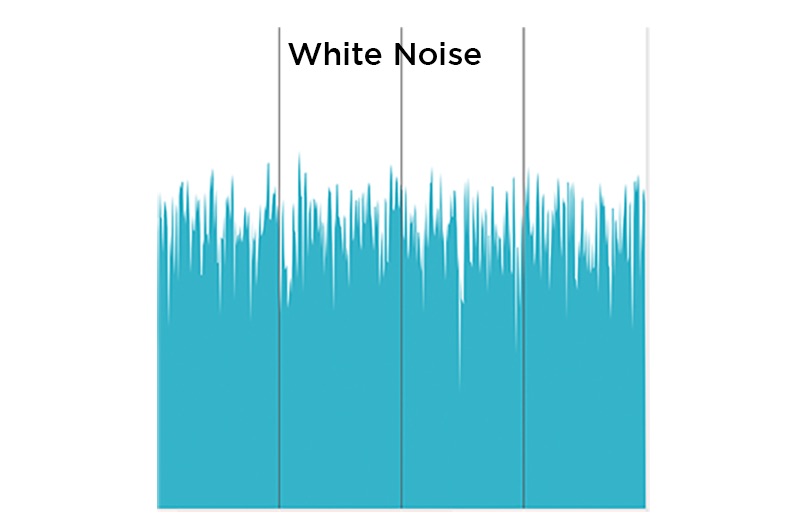
White noise is the most common form of noise that you'll run into. White noise can be seen as the opposite end of the harmonic spectrum from a sine wave. A sine wave has a single frequency component: a fundamental harmonic with absolutely no overtones. If dissected into harmonic components, white noise, on the other hand, contains every frequency, amplitude, and phase relation of a sine wave throughout the audible spectrum. It is sometimes referred to as flat noise as it has equal energy distribution and when plotted on a graph, white noise appears flat and equal. With white noise, all frequencies contain equal amounts of energy. The amount of energy that is contained between 50 and 60Hz is the same as the interval between 500 and 510Hz, as both intervals cover a 10Hz range. Although it is often said to be completely flat and equal, humans hear on a logarithmic scale—meaning that the higher frequencies in white noise are more pronounced to our ears than the lower ones. So despite a technically balanced energy distribution across the entire spectrum, raw white noise often sounds a bit harsh and bright.
Analog white noise can be generated using the thermal noise inherent in vacuum tubes, diodes, or transistors. These are polarized components, meaning they only allow electricity to flow in one direction. By applying a high enough voltage in the opposite direction, stray electrons will occasionally discharge from these components and crash into each other. The resulting noise is extremely low in amplitude and must be amplified considerably. But there you have it, white noise. Can you hear it hissing? White noise is often used as a base for generating other noise types, filtering and redistributing the energy to different frequency spectrums.
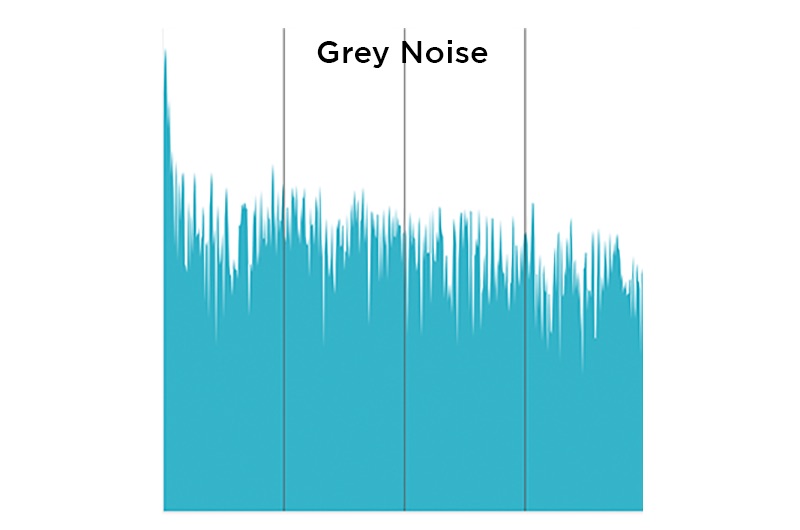
Grey noise is a particularly fascinating form of noise: it is white noise with a psychoacoustically-balanced equal loudness curve over a given range of frequencies. This differs from white noise, which has all frequencies with equal energy. Remember how we said that, because of its technically equal energy distribution, white noise still appears relatively bright and harsh rather than acoustically balanced? Grey noise attempts to counteract our logarithmically-biased hearing, de-emphasizing the harsher aspects of white noise with a specially-designed weighting of different frequencies.
This weighting gives the perception that grey noise is equally loud at all frequencies. It can be thought of as notch-filtered white noise, where the filtering corresponds to the frequency bands most commonly observed by humans. These critical bands of hearing conform to the Bark scale, named after Heinrich Barkhausen by Eberhard Zwicker. The Bark scale defines several frequency bands whose boundaries roughly correspond with humans' capacities for perceiving separation between tones—making it ideal for creating a cleanly-shaped form of perceptually-balanced noise. The Verbos Bark Filter is a fixed filter bank whose frequency bands correspond to the Bark scale.
Darker Noise: Pink and Red Noise
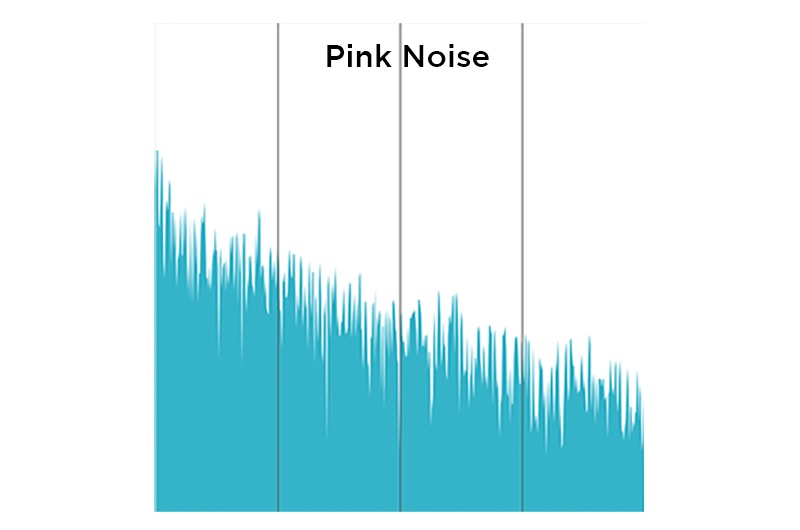
Aside from white noise, pink noise is the most common type of noise used in synthesis contexts. Pink noise is sometimes described as the most pleasant noise to listen to, and is often used in "white noise" machines instead of actual white noise. The spectral power density, when compared to white noise, decreases by 3dB per octave. This means, pink noise can be created by filtering white noise with a filter whose slope is -3dB/oct. This can be sometimes difficult to achieve as there are no simple filters that have that slope, but there are some workarounds. A one pole filter has a slope of -6dB/oct, so you would need a "half-pole" filter in order to create perfect pink noise, which isn't really a thing. Instead of one filter, several filters can be linked in series to create the desired filter slope and a rough approximation of pink noise.
Pink noise has the power per frequency interval that is inversely proportional to the frequency of the signal, which can be expressed as 1/F. When plotted on a graph, it slopes down with the concentration of energy skewed to the lower ranges. Pink noise is sometimes referred to as musically flat noise. Doubling or halving the frequency carries an equal amount of noise energy. So the energy between 50 and 60Hz is the same between 5000 and 6000Hz, as they are equal musical intervals. Each octave in a western musical scale contains the same amount of energy as the octave above and below it, despite covering a wider frequency range. Going an octave up in a musical scale doubles the frequency of the notes in hertz. So the distribution of power is equal across all octaves, hence musically flat noise.
The statistical distribution of 1/F pink noise is often found in nature, man-made systems, and within electronic devices. It is seen within the movements of sandpiles or landslides. The contractions of the heart's muscles have an approximate power distribution of 1/F. Neurons in the brain often have random fluctuations in the ion channels of cell membrane which also follows the statistical fluctuations of pink noise. It is also often found in the statistical distributions found in nature such as water levels, quasar light emissions, and some researchers even say it can be used for predicting the effects of climate change.
Below pink noise in terms of energy distribution is red noise. Red noise may refer to any system where power density decreases with increasing frequency with the power density equal to -6dB/oct or 1/F^2. Red noise is often referred to as brown noise. The term Brown or Brownian noise doesn't actually have to do with the white light/noise analogy, but rather Brownian motion, coined by Robert Brown.
When used as a control or data source, red noise can produce a random walk or drunkard's walk effect, modeling the behavior of molecules suspended in a gas or liquid solution. It can be achieved by randomly adding or subtracting a small fixed value to the previous value. Brownian noise differs from the other noise types as the upcoming states of the noise are informed by the previous states of noise. Strictly speaking, Brownian noise has a Gaussian probability distribution, while red noise can refer to any signal with a -6dB/oct roll off or 1/F^2 frequency spectrum. It has a steep slope and has its energy primarily located in the lower frequency range. Out of the noise types, it arguably performs best as a modulation source on its own. Its low frequency content allows it to modulate parameters without sounding like a constant hissing tone.
Brighter Noise: Blue and Violet Noise
Blue or azure noise has a power density of +3dB/octave, with the density proportional to the frequency over a finite frequency range. It can be thought of as the opposite of pink noise, but favoring higher frequencies over lower ones.
Frequency and energy increase at the same rate with blue noise, so a given octave of blue noise has as much as energy as the two octaves below it combined. Blue noise-type behavior can be observed in the natural world in Cherenkov radiation, where power density increases linearly with frequency. Not only does it reflect the energy distribution of blue noise, but it actually appears bright blue when viewed (pretty cool, right?). Research has shown that retinal cells that form in a distribution similar to blue noise have been observed to correspond to increased visual resolution.
Violet noise (sometimes referred to as purple noise) has a power density that increases 6dB/oct with the density proportional to f^2 over a finite frequency range. It can be generated as a result of differentiation of a white noise signal. You can think of violet noise as opposite to red noise, containing primarily high-frequency content, whereas red noise contains primarily low-frequency content.
The acoustic thermal noise of water has a purple spectrum, which shows up commonly in underwater microphones. Violet noise can be particularly useful for creating hi-hat-like sounds, as it has such a steep low-end roll-off that it can easily sit in a mix without filtering or EQing: simply add an envelope and VCA and you've got a great source of percussive sound.
Other Types of Noise
There are a few other interesting categories of noise outside these "primary" noise colors with a few different definitions. Some of these are fairly standard, and others a bit less commonly referenced—but all are certainly interesting.
Metallic noise is created by mixing or otherwise combining several harmonically rich oscillators together that are tuned with a common fundamental that is below 16Hz (the lower boundary of human pitch perception). This causes the listener to perceive a lack of definite pitch even though it is composed of pitched oscillators. This technique can be heard on the cymbal sound of many early analog drum machines such as the TR-808. It uses six square wave oscillators with the lowest common denominator of pitch at approximately 10Hz, creating a fundamental below the range of human hearing. This is further emphasized by a high pass filter. Metallic noise can be created by combining a several oscillators through mixing or logic, or modules such as the Verbos Random Sampling or Doepfer A-117 come with metallic noise outputs.
So-called orange noise features a finite number of small bands of zero energy. These portions correspond to the frequencies of musical notation and leave only non-harmonically related noise frequencies intact. In other words, it's reverse-quantized noise where the only frequencies that are eliminated correspond to musical notes. It is said to sound particularly inharmonious, even compared to other kinds of noise. To our knowledge, there aren't any commercially available sources of orange noise...but it's certainly an interesting concept.
Green noise has a few definitions. It has been described as a bit like pink noise but its energy is concentrated in the area of 500Hz. It is sometimes used to refer to vocal spectrum noise that is used to test audio circuits. According to some, it represents the ambient sounds of nature, without human intervention. It can also be thought of as bounded brown noise. As with orange noise, the "green noise" designation is mostly novel—though you will run across this term from time to time.
Black noise is defined differently by different schools of thought. One is simply silence, the absence of noise. Another school of thought defines black noise as an active noise suppression system that cancels out noise. Another definition of black noise is a frequency spectrum that is primarily constructed of zero energy zones with occasional spikes of energy. Ultrasonic white noise, above human hearing, is also sometimes referred to as black noise.
Digital Sources of Noise
Things like diodes and transistors that can be used to generate thermal noise in the real world don't exist within software. Therefore, noise has to be generated in a digital environment using different means. Most commonly, this comes in the form of linear feedback shift registers, which act as pseudo-random number generators. This is covered more in-depth in our Learning Synthesis article about randomness, but basically, an LFSR has a clock input which determines its speed and a data input, which is used to seed binary data into the register. The data in this kind of shift register is digital or binary, meaning it has only two states, on or off. A shift register moves the state of the data input into a series of stages and on successive clock events the data in the stages are linearly shifted through the register. Multiple shift register stages, called taps, are XOR'd together and fed into the data input on the shift register. XOR means the data will be active if one or the other taps is high, but low if both or neither are.
A maximal shift register configuration indicates the longest length of a pseudo-random pattern. Different combinations of taps result in different lengths of pseudo-random sequences. The maximum length of a shift register is 2^n-1, or 2 to the power of the step length, minus one. It is minus one because a LFSR state of all stages off is invalid and will result in the LFSR ceasing to work.
An eight-bit shift register has a maximum length of 255 steps. At low frequencies, this will sound fairly random, but when clocked at an audio rate, a definite pattern emerges. Although shorter LFSRs have easily discernible patterns that can be audible to the listener, a maximal-length LFSR with a step length of 48 can be clocked at 96kHz and won't repeat for over 90 years.
The individual stages of the shift register can be combined together using a digital to analog converter which turns the binary signals into discrete values or numbers. Or individual tap outputs can be used to make binary noise. This results in a noise that is crunchy and reminiscent of Atari video games.
Binary noise can also be achieved in a modular synthesizer by feeding a noise source into a comparator. A comparator has a threshold voltage level and when a signal crosses it, the output goes high. It also only has two states, on or off. This is sometimes used in a non-LFSR shift register-based random voltage generator and feeds the data input on the shift register. This can be seen in the Wiard/Malekko Noisering and Music Thing Modular Turing Machine.
Noise Applications
How can noise be used? Well, aside from its obvious sonic applications, the most popular implementation of noise within a modular synthesizer is as a companion to a sample and hold module—in fact, this application is so common that often, noise sources are even included within sample and hold modules. A sample and hold has a CV output and two inputs, a clock input and a sample input. When it receives a clock signal, it looks at the current voltage level of the sample input and takes a snapshot of the voltage level. It takes that voltage level and produces it at the CV output. That voltage level stays constant until the sample and hold receives another clock signal, at which point, the new input voltage is sampled and held. You can see how noise would be a popular choice as a sample and hold source: it allows the creation of random voltages, which can be used to control an oscillator's pitch, a filter's cutoff frequency, and so much more. Different colors of noise offer different results of randomness, with the voltages concentrated where the noise has the most energy.
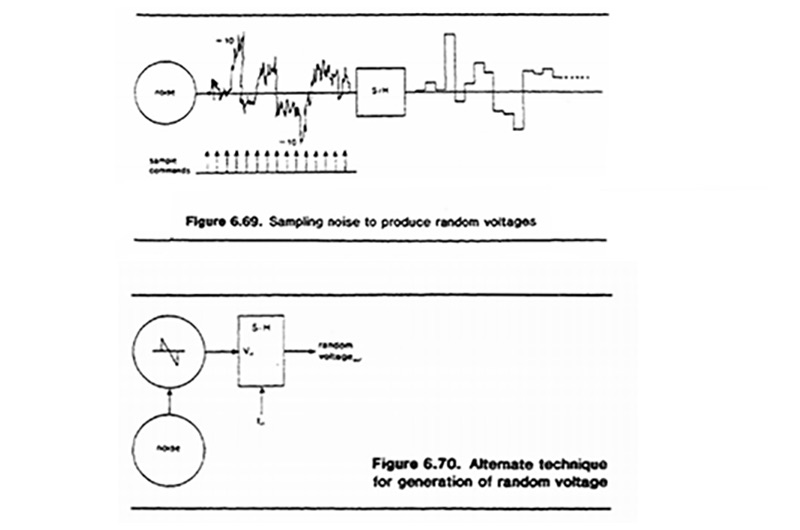
Noise often has a gaussian or bell shaped curve to the statistical probability. This means the majority of the voltages are centered around the middle of the voltage range. One method of changing the probability distribution for the noise into sample and hold technique is to incorporate an oscillator in the signal path. Syncing a sawtooth or triangle oscillator to the noise source will cause changes to the frequency and amplitude of the oscillator to randomly occur at audio rate. This provides a more even distribution of voltage levels with low, medium, and higher range voltages to have the same statistical probability. Using a square or rectangle waveform will result in randomly changing binary outputs, with only two voltage levels. Now, this is assuming a perfectly square wave and perfectly accurate sample and hold. In the real world, occasionally there will be middle voltages in between the high and low points of the oscillator that the sample and hold will hold at. But using a noise-synced square wave into a sample and hold could be another source of binary noise if you'd like.
Noise is often available on synthesizers as an audio source. It can be mixed in with the other waveforms to create more harmonically rich waveforms for the filters to sculpt. This is usually white noise, but pink noise is sometimes available as an option. It is often used for adding an inharmonious element to a sound for applications such as percussive hits or for sound design. An integrated noise source can be seen on synthesizers such as the Minimoog, SH-101, and ARP Odyssey. The noise used for audio is often also used in conjunction with a sample and hold, and sometimes is even available as its own modulation source.
Noise Worth Knowing: Historical Noise Sources
Noise has long been a part of the Buchla family of modular synthesizers, starting with the Buchla 160. The 160 simply had a white noise generator labeled on the top of the unit. Below that, four output jacks with flat and 1/f labels were present. Presumably this refers to white and pink noise. The subsequent 265 and 266, both called the Source of Uncertainty, each had three groups of noise outputs. These were white noise, integrated white noise, and reciprocal white noise. Reciprocal white noise sounds like low pass filtered pink noise, but rather than band limiting the noise, the energy is redistributed. Buchla used a noise-synced triangle wave as the source for the sample and hold modules found on the fluctuating random voltage section on the 265 and 266 and stored random voltage section on the 265.
On the Moog modular, the 903A provided both white and pink noise outputs, with two outputs for each noise type. Additionally, the 923 provided white and pink noise. One added benefit of the 923 was the addition of high pass and low pass filters, which could be used to shape the spectral content of the different noise types.
Also worth noting: the noise source on the classic semi-modular synthesizer, the ARP 2600 is rather unique. It has continuously variable noise color from red to white as well as a level control. It was normalled to the sample and hold, but it was also normalled to the FM input on oscillator 3 and PWM input on oscillator 2. This brings a somewhat non-traditional signal flow to the 2600, as this is not normally how noise is used. Noise isn't usually seen as much as a modulation source as an audio source. If you look at the Buchla noise outputs, they're all designated to the audio form factor, using Tini-Jax instead of banana connectors.
Modern Noise Sources
The Doepfer A-118-2 is a smaller version of the Doepfer A-118-1 and provides noise and random voltages. It outputs white noise, colored noise, continuously variable random voltage, and stepped random voltages. The colored noise section features controls for bringing in both red and blue noise, creating a sort of hybrid noise source that has the characteristics of both flavors of noise.
ETHER from Soma Laboratories is a bit different from the other sources of noise that have been covered. It's not strictly speaking, a noise source—rather, it's kind of an anti-radio. Instead of tuning to a specific channel, ETHER picks up all the electro-magnetic interference and radiation present in the world around us. In lieu of generating thermal noise through components, it picks up electrical waves from hertz to gigahertz and allows you to perceive the invisible landscape that pervades the modern world. Depending on where you are and what devices are near you, the resulting output can vary drastically. In addition to the magnetic sensor, it has two leads which can be used to turn anything conductive to an antenna. It is a tool of sonic exploration that allows you to perceive what the world might sound like to a being attuned to electrical waves and radiation.
While we often think of noise as that awful din coming from the construction site across from our work or the rattle of a subway car, noise within a synthesizer exists outside these definitions. It is a multi-faceted source of unpredictability that has a wide range of applications. Noise can sooth us to sleep or make a bass line really gnarly. The behavior of noise types can be found mirrored in the natural world and within the day to day lives of people. Noise allows us to interface with the unpredictability of the universe and harness it for art and music.